Ethernet is being used to move the world’s data, from planes, trains and automobiles to industrial motor control, autonomous vehicles and the Internet of Things (IOT). The demand to create systems of systems has never been greater, and brings in new challenges to development engineers. They need to gain knowledge on new technologies and on techniques for testing in order to design high speed networks for safety-critical signals. Designing a quality product on time and under budget can be difficult if not impossible without the proper expertise. The key to succeed in this challenge is to uncover product defects in early design stages. But how can this be done without all of the other members in the network? The answer is testing using AUTOSAR, OPEN Alliance or Avnu test suites to ensure conformance to industry standards.
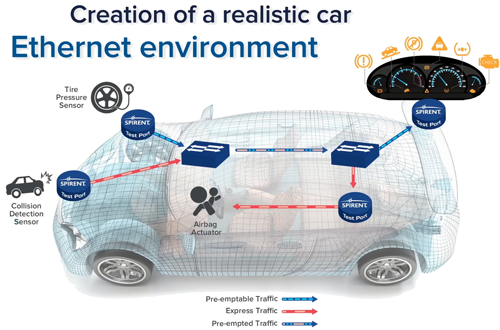
TSN specifications are defining ways to enable zero congestion loss of time-critical data flows. But loss due to congestion is only half of the issue, as network designers and architects also must measure worst-case end-to-end latency within the network. Latency measurements must also consider primary and redundant flows during fault recovery conditions. This is to be able to measure normal vs redundant data path flows in a live network because it is extremely critical to industrial motor control applications. Using the Spirent emulated devices, you can quickly check the Best Clock Master Algorithm (BCMA) inside real devices on the network to ensure they recover from faulted conditions.
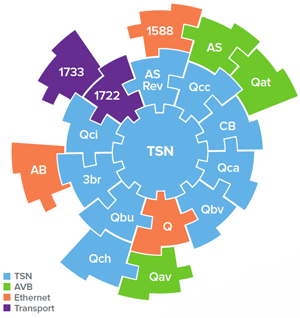
Testing the network with a mix of real devices and Spirent’s Emulated Devices with gPTP functionality will enable designers to find out what scenarios help them to optimize and check network traffic to ensure no loss of time-critical data flows. This is achieved using Spirent embedded counters and timers:
Over 40 measurements tracked in real-time for each received stream including:
Advanced sequencing: In-order, lost, reordered, late and duplicate
Latency: Avg, min, max and short-term avg; first/last frame arrival timestamp
Latency modes: LILO, LIFO and FIFO
Data integrity: Generate Errors: IP checksum, TCP/UDP checksum, frame CRC, embedded CRC and PRBS bit errors
Histograms: Jitter, Inter-arrival, Latency, Sequence
Automated Summary of Timing and Synchronization for IEEE 802.1 – gPTP
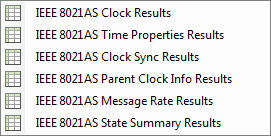
Spirent also automatically calculates the following IEEE802.1as gPTP Clock information:
IEEE802.1as Clock Results
States: Clock Identity, State, Clock Accuracy
Timers: Current, Min, Max, Avg Mean Path Delay
Counters: TX / RX Announce, TX/RX Sync, TX / RX Follow up, TX / RX Peer Delay Request, TX / RX Peer Delay Response, TX / RX Peer Delay Follow up
IEEE802.1as Time Properties Results
States: gPTP Time Scale, Current UTC offset Valid, Leap59, Leap61, Time/Frequency Traceable, Time Source
Counters: Current UTC offset
IEEE802.1as Clock Sync Results
Timers: Time of Day
Counters: Current offset, Positive / Negative offset Peak and deviation, Current, Min, Max, Average Mean Path Delay, Average offset plus / minus deviation, Step Removed, Minimum Pdelay Request Interval, Peer Mean Path Delay, Sync / Follow-up / Pdelay Correction Field Response and follow-up, Invalid Timestamp count
IEEE802.1as Parent Clock Info Results
States: Parent Stats, Step Mode
Timers: Observed Parent offset scaled log Variance, Observed Parent Clock Phase Change Rate, Grandmaster Identity, Grandmaster Clock Class, accuracy, offset Variance, Grandmaster Priority 1, 2
IEEE802.1as Message Rate Results
Counters: Announce Rate: Tx / Rx Min, Max Average Packets per second,Sync Rate: RX Min, Max, Average Packets per second, Follow up Rate: RX Min, Max, Average Packets per second, Peer Delay Request Rate: RX Min, Max, Average, Peer Delay Response Rate: RX Min, Max, Average, Peer Delay Response Follow up Rate: RX Min, Max, Average
IEEE802.1 State Summary Results
Counters: Faulty, Disabled Count, Listening Count, Pre-Master Count, Master / Slave Count, Passive, Uncalibrated Count, 802.1as up / down
Impairment / Error injection - Timing and Synchronization for IEEE 802.1 – gPTP
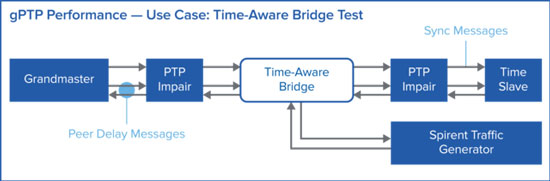
As you are deploying a time-aware network we must take into consideration timing errors. The two main contributors to timing errors are the accuracy of the correction field and the peer delay calculations for each member in the network. Do they reflect the actual delay experienced by the sync messages? Possible errors:
Variable Errors
Quantization errors in time stamps
Synchronization/rounding errors in local DUT clocks
Phase noise in oscillators
Fixed Errors
Asymmetric delays in physical layer ie: time stamp type and point
Cable lengths between forward and reverse paths
In a typical device under test the application functionality turnaround time can and will impact the downstream path delay calculation when internal DUT hardware and software resources are shared between the application functions and network communications.
Spirent provides a test bed that emulates, measure and impair gPTP networked devices using industry proven techniques and products to help develop robust products with accurate timing.
Want a step by step guide to conducting the right tests?
Get the full Time-Sensitive Networking Automotive Playbook
Or learn more about our automotive testing solutions here: